The unit of time (the ‘second’) can now be measured more accurately than any other physical quantity and there has been a tenfold increase in the accuracy of the measurement of time and frequency in each decade since the end of the Second World War. None of this progress would have been possible without the atomic clock. Sixty years ago, the invention of the atomic clock enabled the International Bureau of Weights and Measures (BIPM) to change the unit of time from one based on the solar system and astronomy to a definition based on the atom and physics. Atomic clocks are the most accurate timekeeping devices in practical use today but for how long? With much apprehension, I will put my head above the parapet and try to answer that question.
Atomic clocks are used by every sector of society and what started as a tool for the military is now common-place in the financial, industrial and consumer markets. Precise timing is needed for applications ranging from missile control to banking, secure communications to air traffic management and location-based technologies to in-car navigation and computer gaming.
The atomic clock was made possible through the brilliant theoretical work of a number of scientists in America but the first, fully operational atomic clock was developed by a British physicist, Louis Essen, in 1955, whilst working at the National Physical Laboratory (NPL) in Teddington, Middlesex, Figure 1.
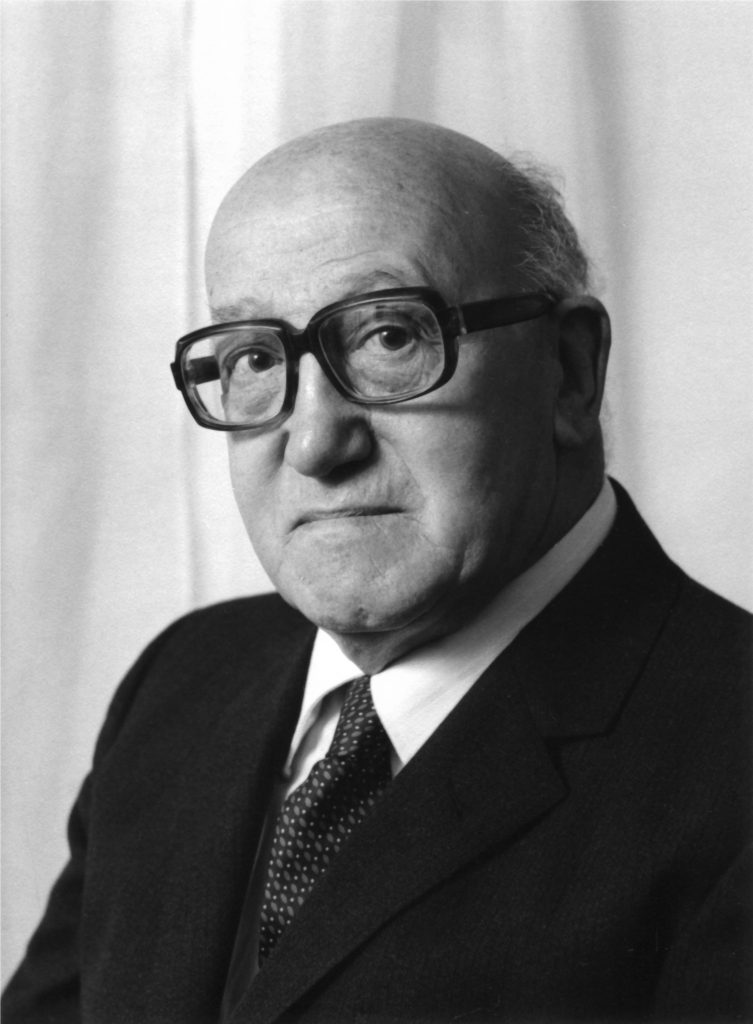
World Time
The search for the ultimate clock is never ending and many inventors have exercised their ingenuity in devising clocks that outperform Essen’s original atomic clock. Improvements have never ceased but, at a fundamental level, the latest research into precise time measurement is still based on the properties of the atom rather than heading towards an entirely new concept.
Scientists are still looking at ways to refine the accuracy, precision and reliability of atomic clocks. The most advanced clocks in 2021 have an accuracy equivalent to 1 second in 300 million years. This is a huge achievement and a significant development since Essen’s first atomic clock, Figure 2. Yet, all of today’s innovation is based on the natural properties of atoms or ions (atoms that carry an electric charge), exactly like Essen’s first atomic clock.
Scientists are still looking at ways to refine the accuracy, precision and reliability of atomic clocks. The most advanced clocks in 2021 have an accuracy equivalent to 1 second in 300 million years. This is a huge achievement and a significant development since Essen’s first atomic clock, Figure 2. Yet, all of today’s innovation is based on the natural properties of atoms or ions (atoms that carry an electric charge), exactly like Essen’s first atomic clock.
NPL is one of around 80 scientific institutes that maintain the international time scale, Coordinated Universal Time (UTC). This is the basis for all precision time measurement as well as civil timekeeping. UTC is derived by averaging around 400 atomic clocks from around the world, including those at NPL.
At present, the national UTC time scales of different countries are linked either by exchanging radio signals via communication satellites or by comparisons using the signals from atomic clocks onboard navigation satellites. Optical fibre links promise a substantial improvement in the accuracy of international time scale comparisons in the future.
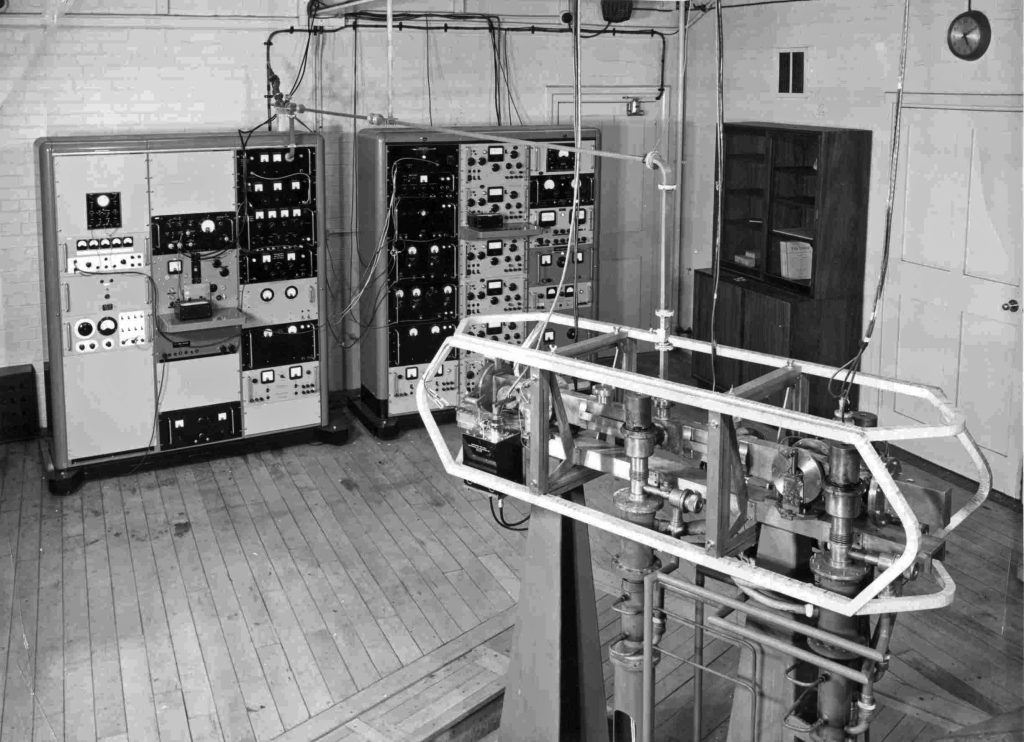
UK Timing Centre
There is a saying, attributed to the Canadian media critic Marshall McLuhan: ‘We shape our tools and then our tools shape us.’ The invention of the atomic clock and the birth of atomic timekeeping were shaped by the demands of the Cold War superpowers for better navigational systems. But within a few decades, atomic clocks started to play an increasingly important role in our daily lives. And the combined forces of atomic clocks, the internet and electronic gadgets are reshaping today’s society, making what was once the preserve of a few scientists something that has implications for all of us.
The world is becoming more and more reliant on the US-operated Global Positioning System (GPS) for navigation and timing information. Britain’s emergency services, power distribution networks and the economy are particularly dependent on GPS and other similar satellite-based systems. A failure of the GPS system has been identified as a major risk to our country. That is because all global navigation satellite systems (GNSS), including GPS, are based on weak, low-power radio signals that are vulnerable to interferences such as jamming, spoofing and solar storms.
The UK government has responded by pledging to fund a National Timing Centre that will oversee a new, dedicated network of atomic clocks, which will free the UK from its dependence on precise time sources controlled by foreign agencies. In the event of failure or lock-out, a network of atomic clocks will act as a local alternative to GPS and provide a fully resilient source of time to safeguard the country’s critical infrastructure.
The UK government has responded by pledging to fund a National Timing Centre that will oversee a new, dedicated network of atomic clocks, which will free the UK from its dependence on precise time sources controlled by foreign agencies. In the event of failure or lock-out, a network of atomic clocks will act as a local alternative to GPS and provide a fully resilient source of time to safeguard the country’s critical infrastructure.
The National Timing Centre is planning to deliver its services via a network of underground fibre-optic cables. An alternative method of providing precise time and location services in future may be to create a mega-constellation of small satellites in low-earth orbit, just 1,200 kilometres high. The satellites would be in a much lower orbit than the current generation of global navigation satellite systems which orbit the earth at a height of approximately 20,000 kilometres. This means that the high-power signals would be less susceptible to jamming and the system could provide a more resilient alternative to GPS and other similar systems such as the European Galileo project. This is still unproven technology but it offers the possibility of piggybacking internet and voice telephony services onto the constellation of small satellites.
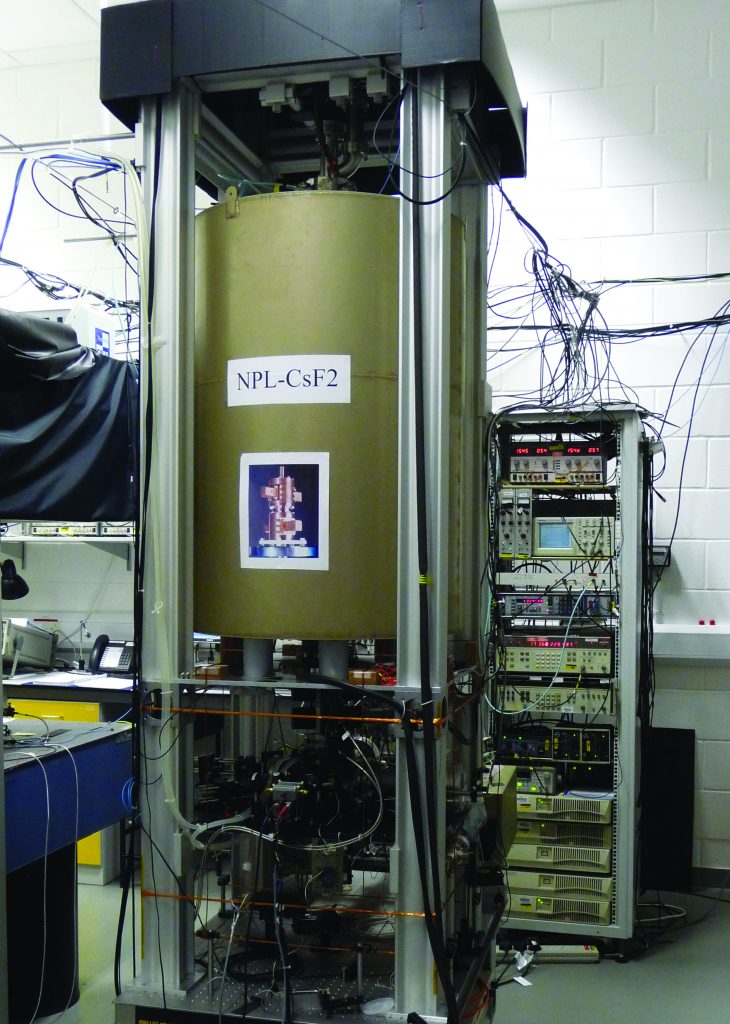
Think Small
A branch of research that is becoming increasingly important is the miniaturisation of atomic clocks. Researchers have already begun to develop new techniques for the mass production of low-cost, chip scale atomic clocks with the goal of producing a one-cubic-centimetre device that has a high degree of accuracy and reliability, coupled with low power consumption. New applications and technologies like 5G mobile communication networks are driving much of this demand. Highly accurate time synchronisation will be needed across the network if 5G is to realise its full potential.
Smaller versions of the current generation of atomic clocks could replace quartz crystal oscillators which are commonly used in computers and battery-powered handheld devices such as smartphones for military use. The US Defence Advanced Research Projects Agency (DARPA) is already working on an advanced version of its first-generation, chip scale atomic clock for use in applications such as navigation, timing and battlefield communications.
Optical Clocks
To achieve the goal of developing miniature atomic clocks, scientists are experimenting with atoms and ions that have suitable spectral lines in the optical region, rather than the microwave region – which was used for the first generation of atomic clocks. Optical waves (light) have a frequency that is five orders of magnitude higher than that of microwaves. These higher frequencies are being used to probe narrow optical transitions in atoms or ions, which could lead to the development of devices that have a performance that is far higher than the current generation of atomic clocks.
Early atomic clocks relied on different atoms for different applications. For instance, caesium clocks have excellent reliability and stability; and the ‘second’ is defined in terms of caesium in the international system of measurement (SI). Hydrogen is currently the best choice of atomic clock in terms of its short-term frequency stability but hydrogen masers are relatively large, complex and expensive devices. On the other hand, rubidium atomic clocks are small, lightweight and relatively cheap. They also have a long life with adequate performance, which makes them a good choice for use in satellites. Scientists are investigating a range of different chemical elements for use in optical clocks which are harder to build but offer the prospect of better overall performance.
There are currently two main types of optical clock: those based on ‘ion traps’ and those based on ‘optical lattice traps’. Ion traps contain single trapped ions of chemical elements such as strontium or ytterbium, which are cooled by lasers until their temperature is close to absolute zero (273 degrees below the freezing point of water), when the ions become almost stationary. These ions are then trapped by electromagnetic fields almost indefinitely. Optical lattice clocks trap cooled atoms using two or more counter-propagating laser beams. Atoms are chosen based on their ability to be cooled as well as for their narrow electronic transitions. The most commonly chosen atom for this type of clock is the metallic element, strontium, Figure 4.
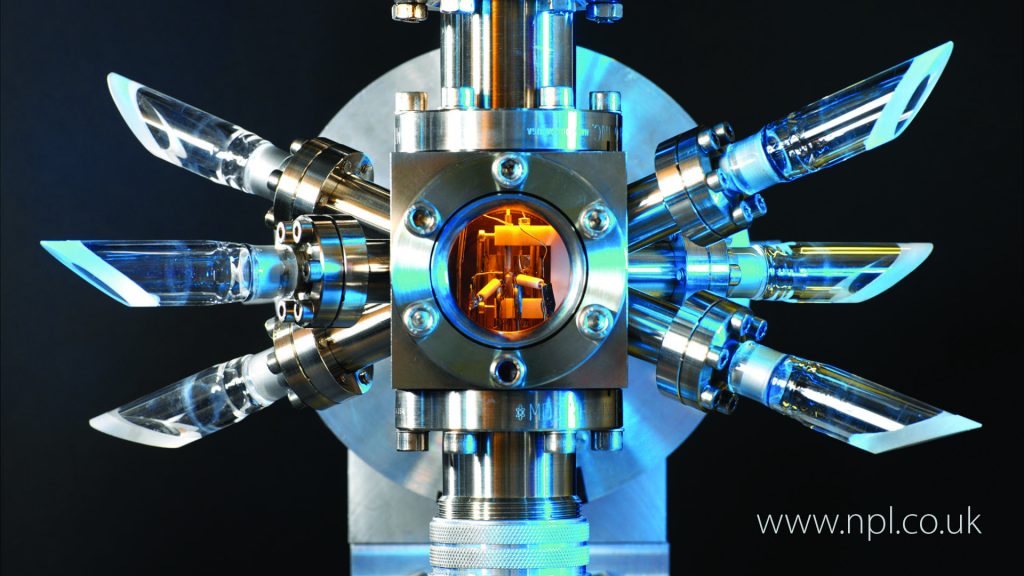
There is another group of atomic clocks with a promising future. These are ‘quantum logic clocks’ which use the same principle as ion traps but they contain several ions rather than one. For example, aluminium ions have appropriate properties but are difficult to cool to the required temperature so they are combined with magnesium ions which can be cooled more easily. These clocks may ultimately have accuracies around a hundred times higher than current atomic clocks. This gives a much higher performance, equivalent to losing no more than one second in the lifetime of the universe.
Optical clocks have become popular since the development of the femtosecond frequency comb. A ‘frequency comb’ is a laser source whose spectrum consists of a series of discrete, equally spaced frequency lines. These combs enable physicists to measure the frequencies of optical devices against the standard frequency of the caesium atom. In effect, this establishes the exact relationship between the frequencies of optical clocks and microwave clocks. A frequency comb makes it possible to directly count the ‘ticks’ of an optical atomic clock. This is proving to be an essential component in the design and construction of clocks that operate in the visible part of the electromagnetic spectrum.
New Applications
Optical clocks are so precise that they can detect a difference between two clocks separated in elevation (height above sea level) by as little as one centimetre. This is interesting from the standpoint of showing that Einstein’s theory of relativity is correct. It also means that these clocks open new possibilities for scientists who are studying the earth sciences.
Future devices could detect subtle changes in elevation, relative to the surface of the earth. And this could pave the way for the development of new types of measuring devices which could be used to predict the occurrence of volcanic eruptions and earthquakes.
In general, the ticking rate of these next-generation atomic clocks can still be altered by outside influences such as magnetic and electric fields, force, motion, temperature and gravity. But in time, this could also become a benefit instead of a drawback. Their sensitivity to outside disturbances make optical clocks prime candidates for a new range of high performance sensors.
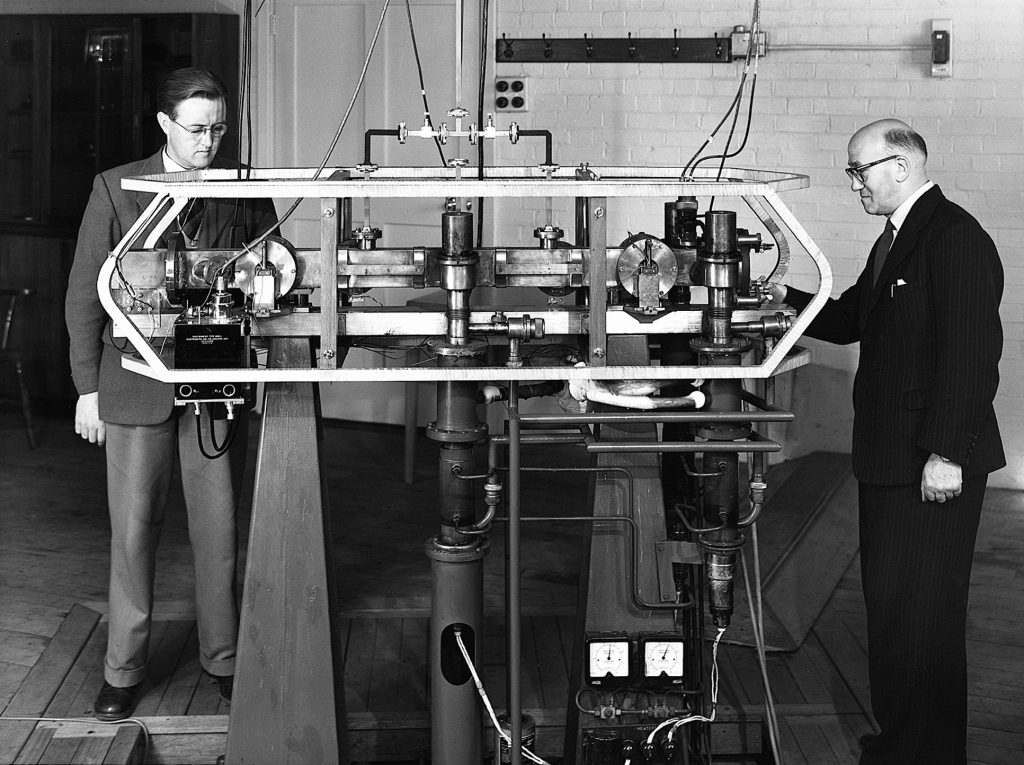
Definition of Time
As optical clocks have evolved, their error margins have become lower than those of caesium-beam atomic clocks. This means that the scientific community is recognising a need to move away from the present definition of the second based on the caesium transition frequency, measured by Essen, and towards a new definition unrelated to caesium.
The current definition of the second, according to the International Bureau of Weights and Measures, is: ‘The duration of 9,192,631,770 periods of the radiation corresponding to the transition between the two hyperfine levels of the ground state of the caesium 133 atom.’ That’s a bit of a mouthful but it just means that a caesium clock will always ‘tick’ at a steady rate of nearly ten billion times a second, Figure 5.
There are several possible candidates for the new second and a final decision is yet to be made. One option is to define the second in terms of a selected optical frequency. While it would be easier to base a definition around a specific atomic frequency of a particular chemical element, deciding which frequency and which element to choose is not an easy task. There is a lot of debate around whether to go for an experiment which has been repeated in several laboratories but may not be quite as accurate, or whether to go for one which has only been done at one laboratory but has a higher accuracy value.
Essen’s legacy still lives on in the clock mechanism that we use to define the unit of time. Atomic clocks remain the order of the day, although much modified for improved accuracy, precision and stability. However, the current definition of the second, based on the caesium transition frequency, is gradually losing its usefulness. A redefinition is still some years away and each of the competing alternatives has its merits.
Time will tell which option the scientific community eventually chooses to recommend in place of the caesium clock, first brought into operation by Louis Essen in 1955.
Figure 1. Louis Essen.
Figure 2. Essen’s first atomic clock.
Figure 3. Experimental fountain clock.
Figure 4. Optical atomic clock. Image courtesy of NPL.
Figure 5. Measuring the frequency of the caesium atom.
About the Author
Ray Essen has written extensively about horology and the work of his late father-in-law, Louis Essen.
His latest book, Revolutions in Time, tells the story of the birth of atomic timekeeping and the impact of Louis Essen’s work on the development of radio, radar, navigation and astronomy. Look out for a review in the next issue of TimePiece.
If you would like to get a copy of the book it is available from Amazon in paperback (£9.99) or Kindle (£4.99) or free if you are a Prime subscriber.
Ray is donating all the royalties to help the mental health charity, Mind.
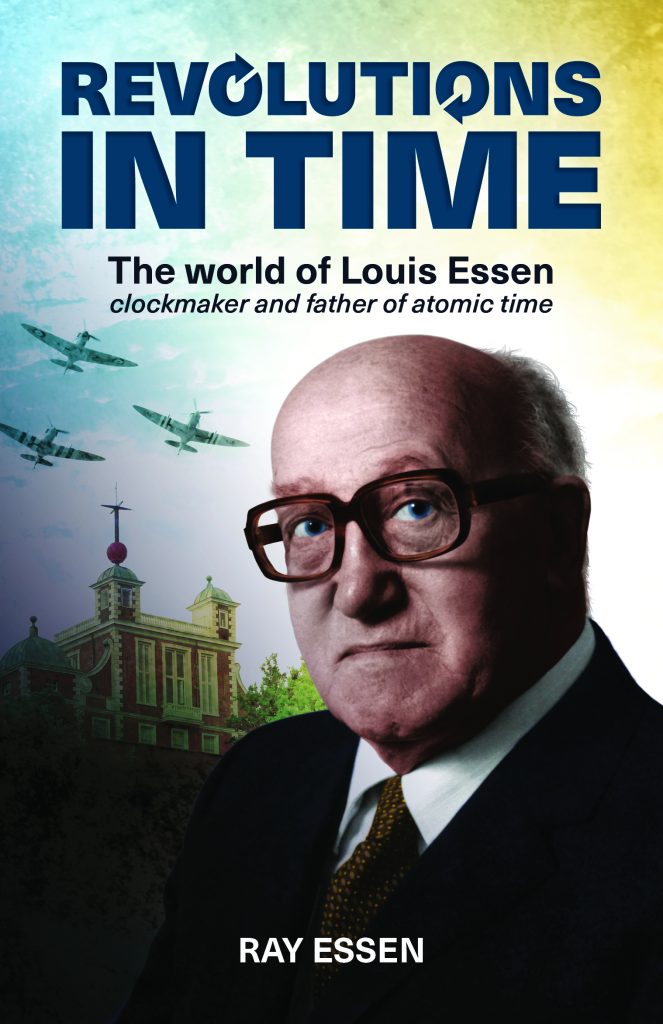
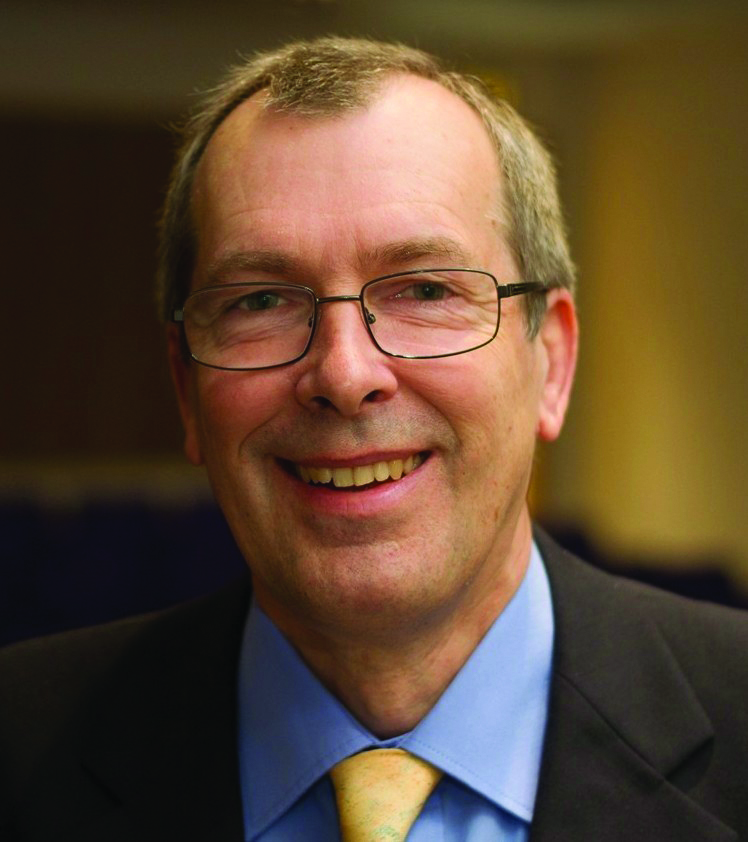